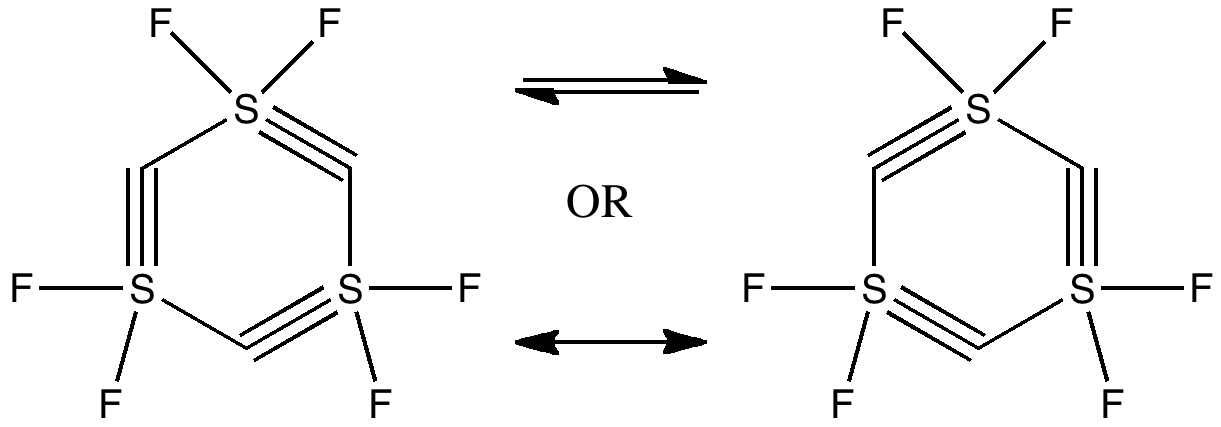
In the previous post, the molecule F3S-C≡SF3 was found to exhibit a valence bond isomerism, one of the S-C bonds being single, the other triple, and with a large barrier (~31 kcal/mol, ν 284i cm-1) to interconversion of the two valence-bond forms.
In the previous post, the molecule F3S-C≡SF3 was found to exhibit a valence bond isomerism, one of the S-C bonds being single, the other triple, and with a large barrier (~31 kcal/mol, ν 284i cm-1) to interconversion of the two valence-bond forms.
A previous post posed the question; during the transformation of one molecule to another, what is the maximum number of electron pairs that can simultaneously move either to or from any one atom-pair bond as part of the reaction?
In the previous two posts, a strategy for tuning the nature of the CS bond in the molecule HO-S≡C-H was developed, based largely on the lone pair of electrons identified on the carbon atom. By replacing the HO group by one with greater σ-electron withdrawing propensity, the stereo-electronic effect between the O-S bond and the carbon lone pair was enhanced, and in the process, the SC bond was strengthened.
In my first post on this theme, an ELF (Electron localization function) analysis of the bonding in the molecule HO-S≡C-H (DOI: 10.1002/anie.200903969) was presented. This analysis identified a lone pair of electrons localized on the carbon (integrating in fact to almost exactly 2.0) in addition to electrons in the CC region.
Steve Bachrach has just blogged on a recent article (DOI: 10.1002/anie.200903969) claiming the isolation of a compound with a C≡S triple bond; A compound with a C≡S triple bond Steve notes that Schreiner and co claim a “structure with a rather strong CS double bond or a weak triple bond”. With this size of molecule, the proverbial kitchen sink can be thrown at the analysis of the bonding.
The Grignard reaction is encountered early on in most chemistry courses, and most labs include the preparation of this reagent, typically by the following reaction: 2PhBr + 2Mg → 2PhMgBr ↔︎ MgBr 2 + Ph 2 Mg The reagent itself exists as part of an equilibrium, named after Schlenk, in which a significant concentration of a dialkyl or diarylmagnesium species is formed.
We have seen in the series of posts on the topic of hypervalency how the first row main group elements such as Be, B, C and N can sustain apparent hypercoordination and arguably hypervalency. The latter is defined not so much by expanding the total valence shell of electrons surrounding the hypervalent atom beyond eight, but in having more than four well defined bonds to it, as quantified by AIM and ELF analysis.
Quite a few threads have developed in this series of posts, and following each leads in rather different directions. In this previous post the comment was made that coordinating a carbon dication to the face of a cyclopentadienyl anion resulted in a monocation which had a remarkably high proton affinity.
The previous posts have seen how a molecule containing a hypervalent carbon atom can be designed by making a series of logical chemical connections.
The previous post talked about making links or connections. And part of the purpose for presenting this chemistry as a blog is to expose how these connections are made, or or less as it happens in real time (and not the chronologically sanitized version of discovery that most research papers are). So each post represents an evolution or mutation from the previous one.