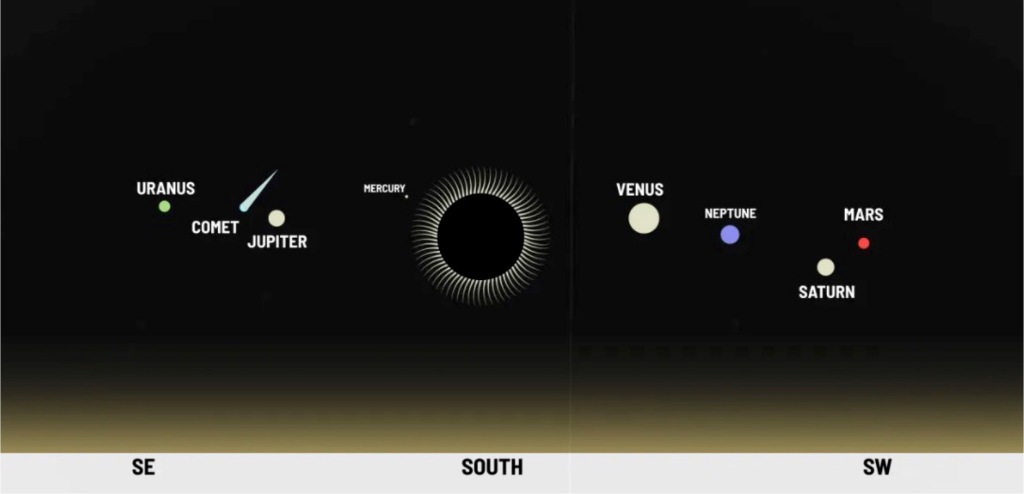
Perhaps the most compelling astronomical phenomenon accessible to a naked-eye observer is a total eclipse of the sun. These rare events have always fascinated us, and often terrified us. It is abnormal and disturbing for the sun to be blotted from the sky! A solar eclipse will occur on Monday, 8 April 2024. A partial eclipse will be visible from nearly every part of North America.